Atomic resolution NMR Diffraction
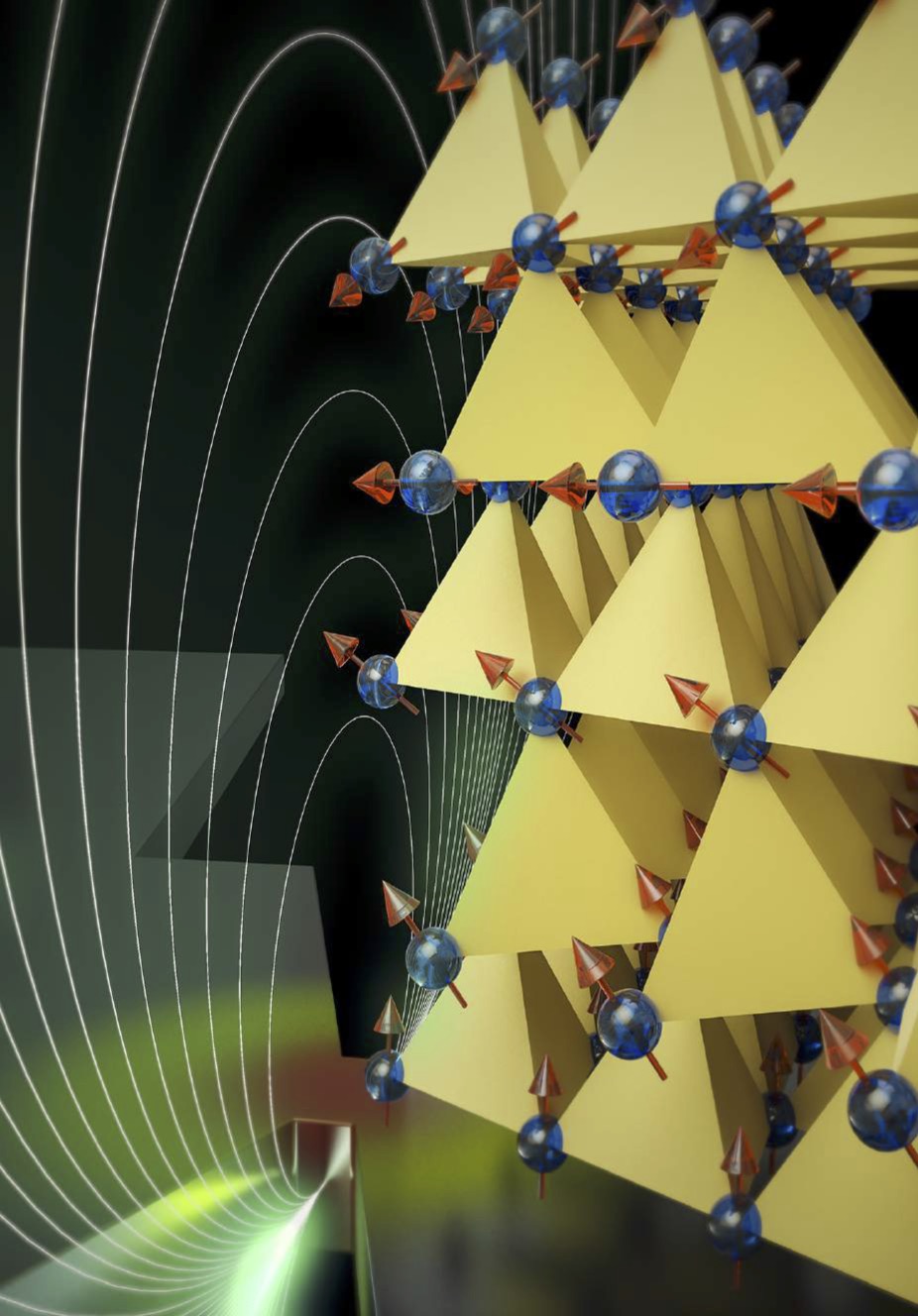
Before the invention of magnetic resonance imaging (MRI), Mansfield and Grannel proposed a new method for probing the structure of crystalline materials through their nuclear spins. This technique, called NMR diffraction (NMRd), would be a revolutionary tool for studying the structure of materials if realized on the atomic scale. Given the spectroscopic capabilities of NMR, and its non-destructive nature, this would have profound implications as a tool for structural biology, including the study of proteins and virus particles. At the time of the proposal, the combination of high field gradients and long coherence times necessary for achieving atomic resolution NMRd was technologically unfeasable. With the recent development of large magnetic field gradient sources, along with high-fidelity quantum control for dynamical decoupling, realizing NMRd on the atomic scale is now within reach.
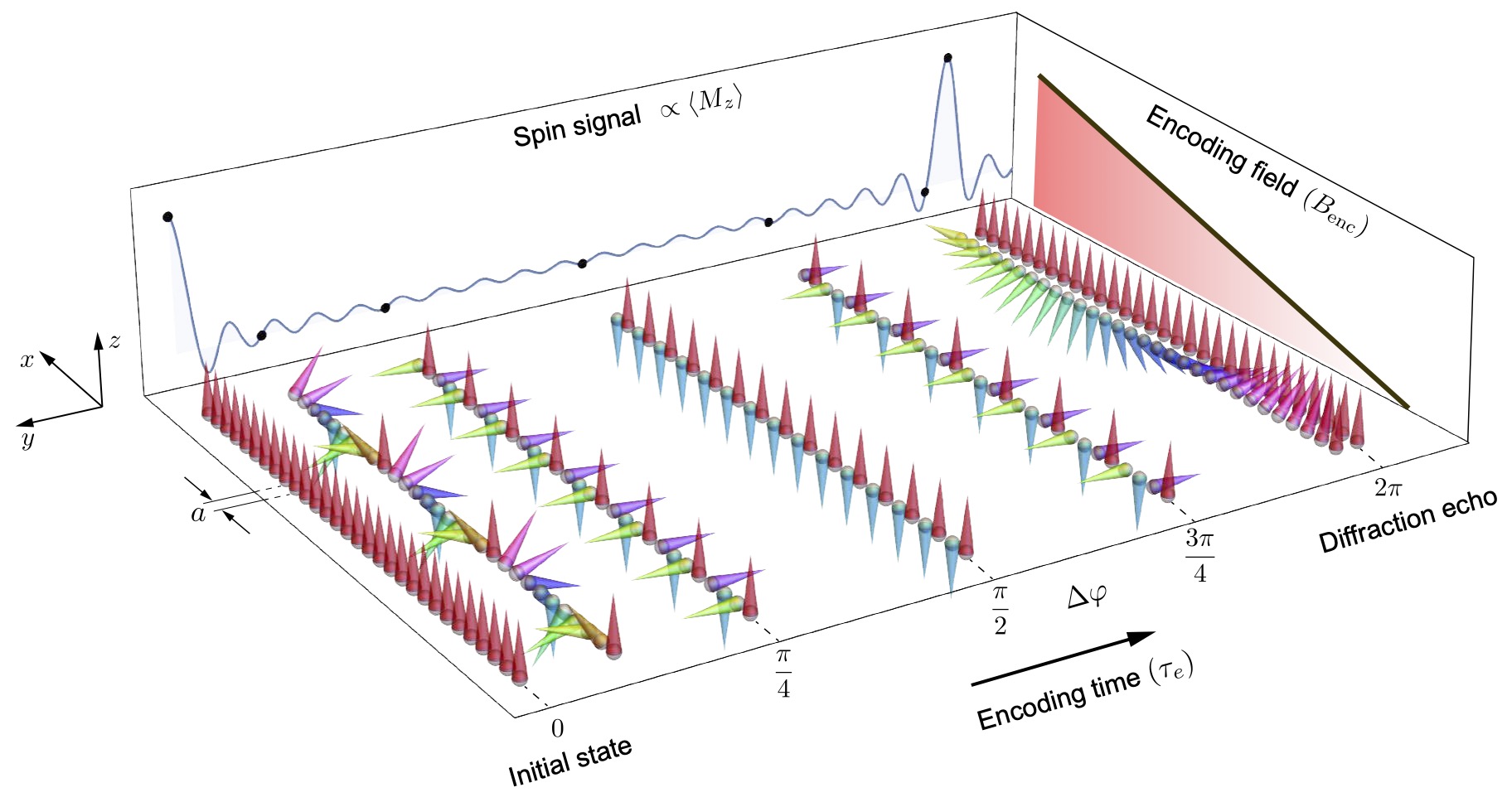
Recently, we demonstrated nanoscale NMRd on of ∼2 million 31P spins in a nanoscale volume of an indium-phosphide (InP) nanowire [1]. This was obtained by encoding a nanometer-scale spatial modulation of the z-axis magnetization and detecting the period and position of the modulation with a sub-angstrom precision. Along with this, we utilized a similar scattering-based method to detect an angstrom-scale displacement of the InP sample with picometer precision. These diffraction-based techniques can extend the Fourier-encoding capabilities of NMR to the angstrom scale and demonstrate the potential of NMRd as a tool for probing the structure and dynamics of nanocrystalline materials.
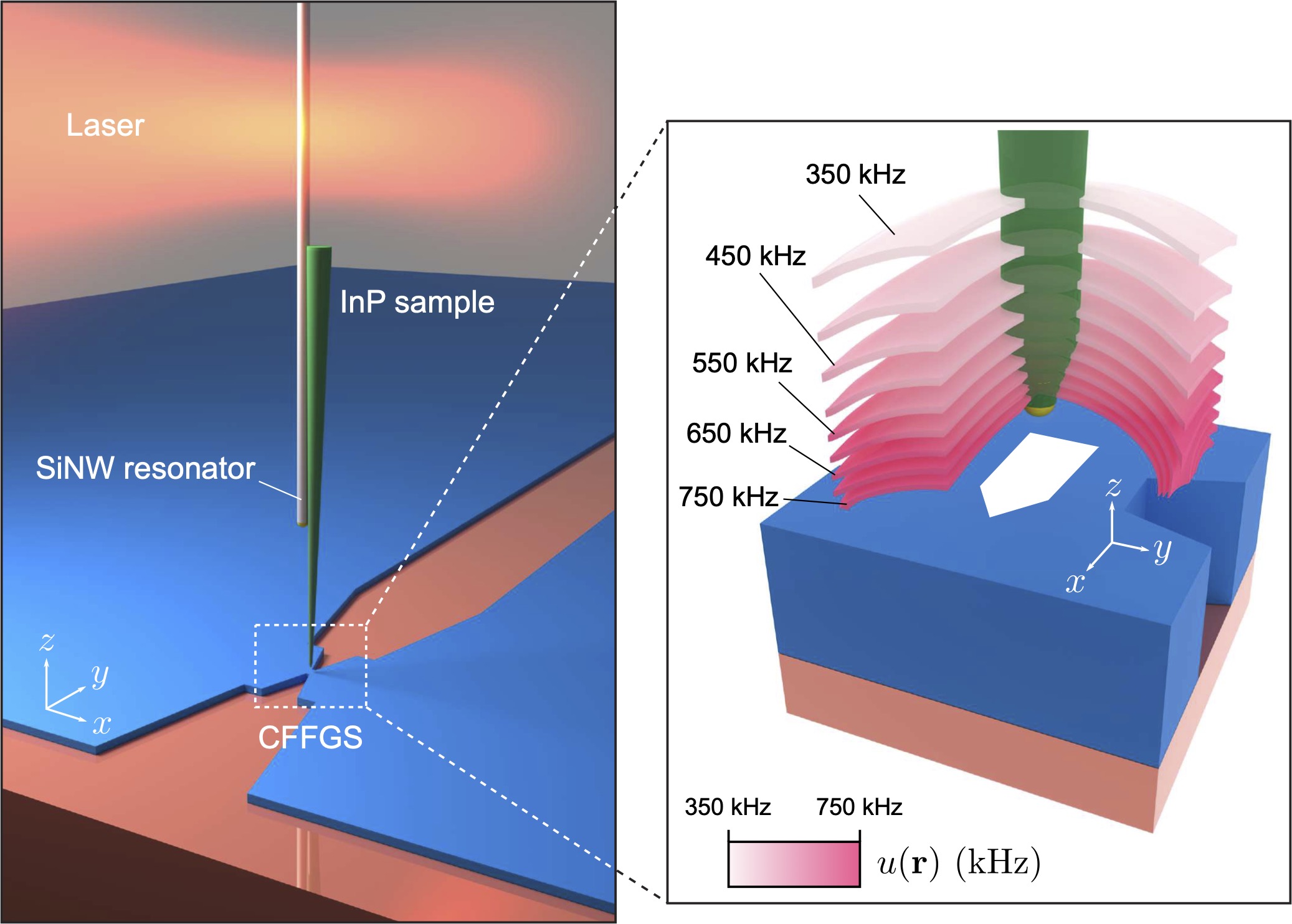
Upcoming experiments will utilize novel gradient source devices to generate highly uniform 3D magnetic field gradients suitable for crystallographic NMRd measurements. One potential sample is Fluorapatite, which is known for its interesting many-body dynamics that approximate 1D spin chains.
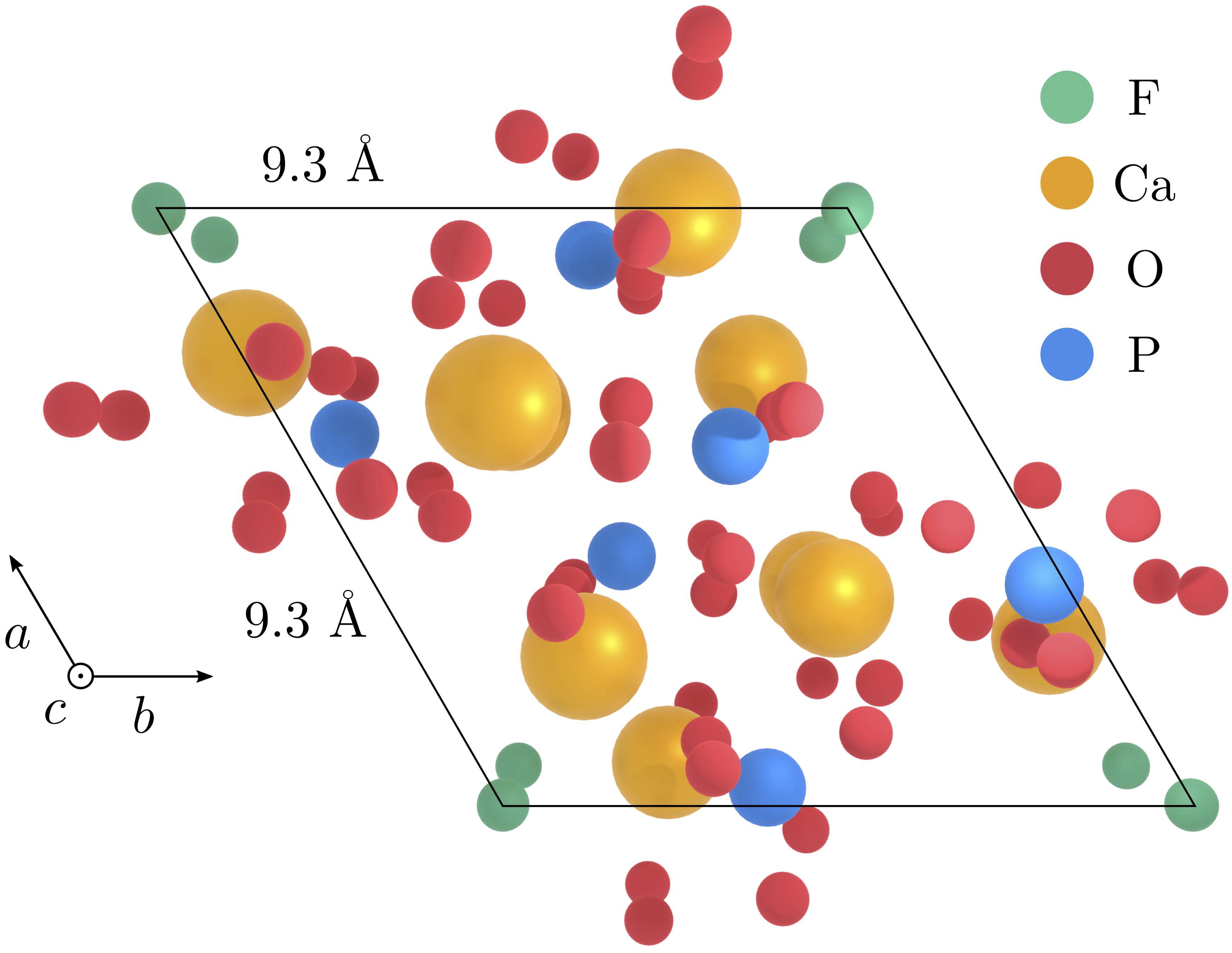
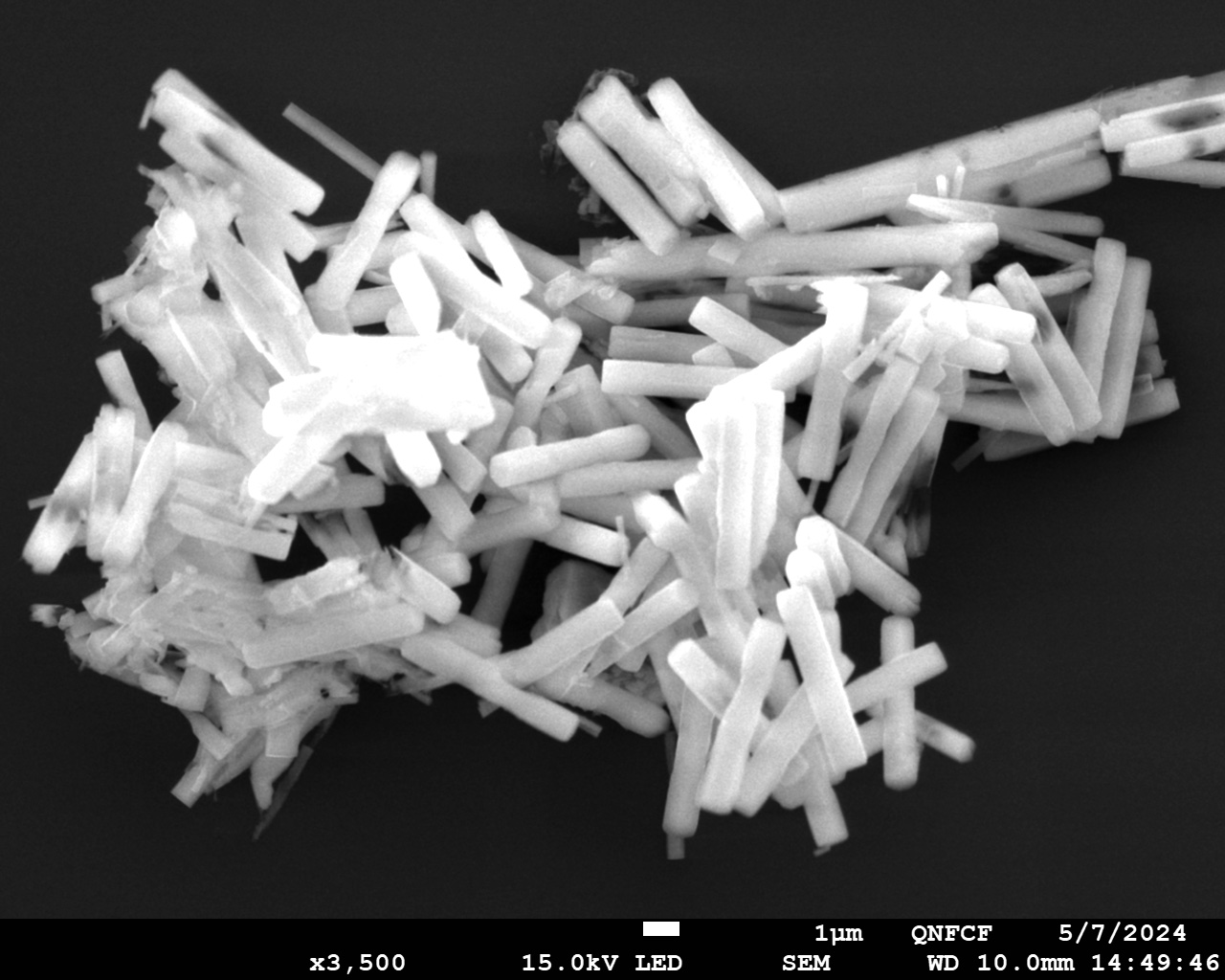