Spin Transport
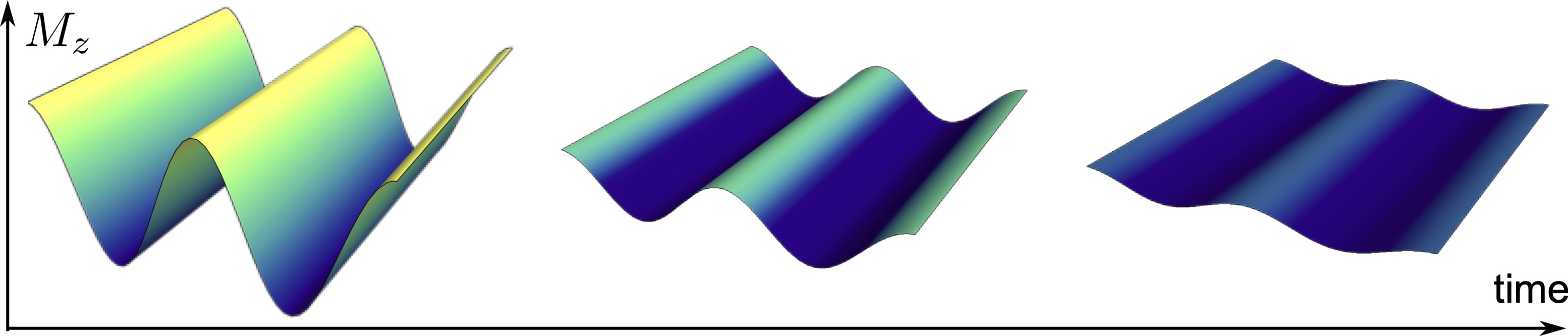
Under a high static field, the local polarization of a dipolar-coupled spin ensemble is known to follow diffusive dynamics on length scales much larger than the lattice spacing. This kind of "spin diffusion", is a well-known many-body phenomenon that has been experimentally studied at micron length scales [1]. The combination of large magnetic gradients in our nanoMRI setup and dynamical decoupling sequences allows us to study dipolar spin dynamics at length scales close to the lattice spacing, and examine the exact boundary between coherent microscopic dynamics and spin diffusion.
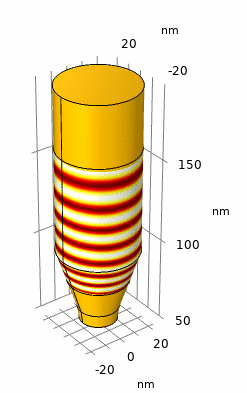
We have demonstrated this using Indium Phosphide (InP) nanowires, which are attached to our silicon nanowire (SiNW) force sensors and positioned above our gradient source device. Using the combination of large field gradients along with dynamical decoupling, the InP nanowire is encoded with a spatial modulation along its c-axis with varying pitches. This magnetization profile is allowed to diffuse throughout the sample under dipolar couplings followed by the application of a decoding sequence, which encodes the correlation between the initial and time-evolved modulations into the measured signal. The time-scale of the spin transport can be determined through this measured correlation.
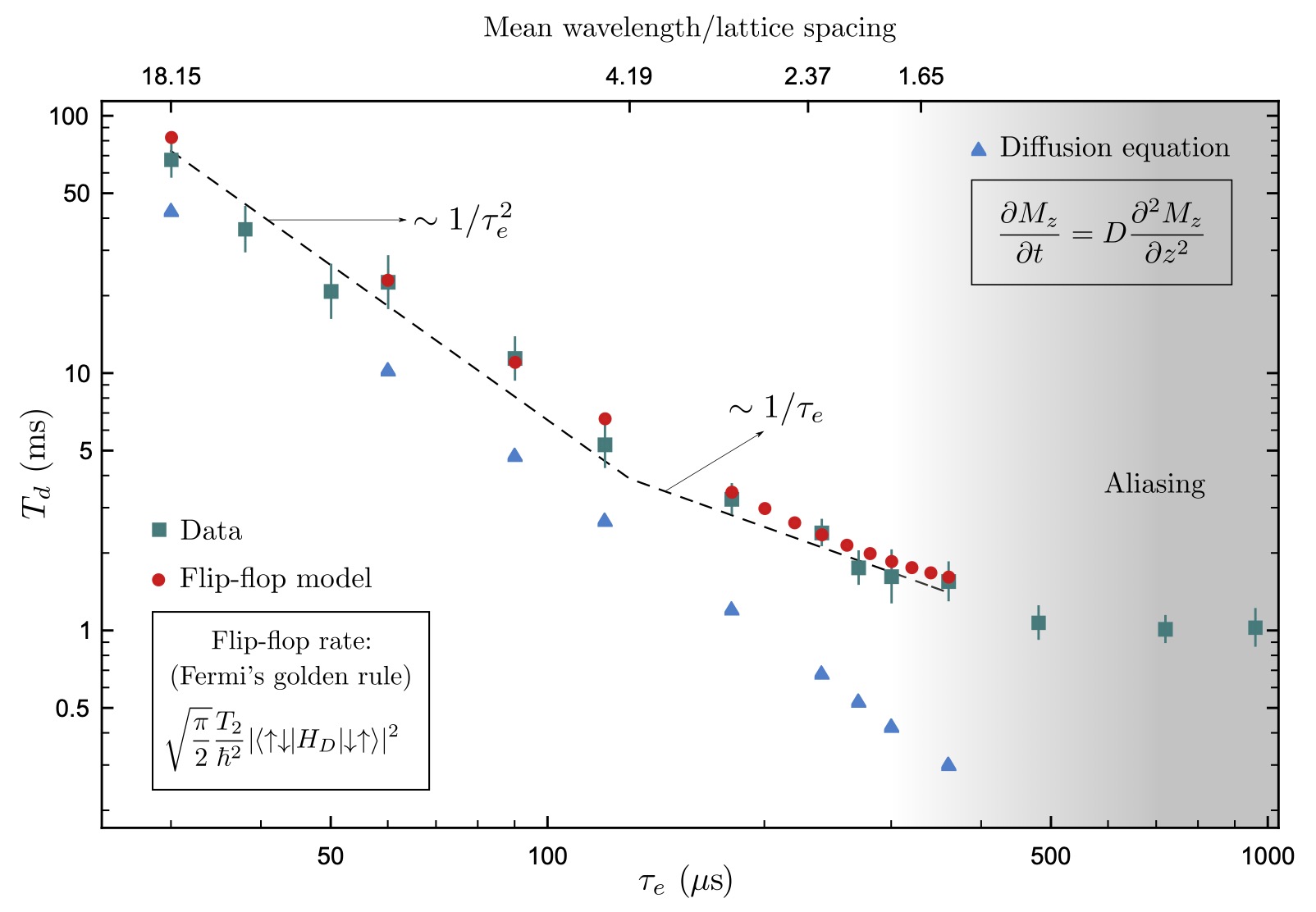
On changing the pitches to ∼4a, where a is the lattice spacing, the diffusive behaviour breaks down and the transport follows a ballistic behaviour i.e., the decay time constant of the z-magnetization follows an inverse law rather than an inverse square law with the spatial encoding frequency. We measured the transport properties of the z-magnetization over different pitches and confirmed this ballistic behaviour. We also performed finite-element simulations of the diffusion equation, along with a semi-classical flip-flop model, analogous to a random walk, to observe the length scales at which the transport deviates from a diffusive behaviour.
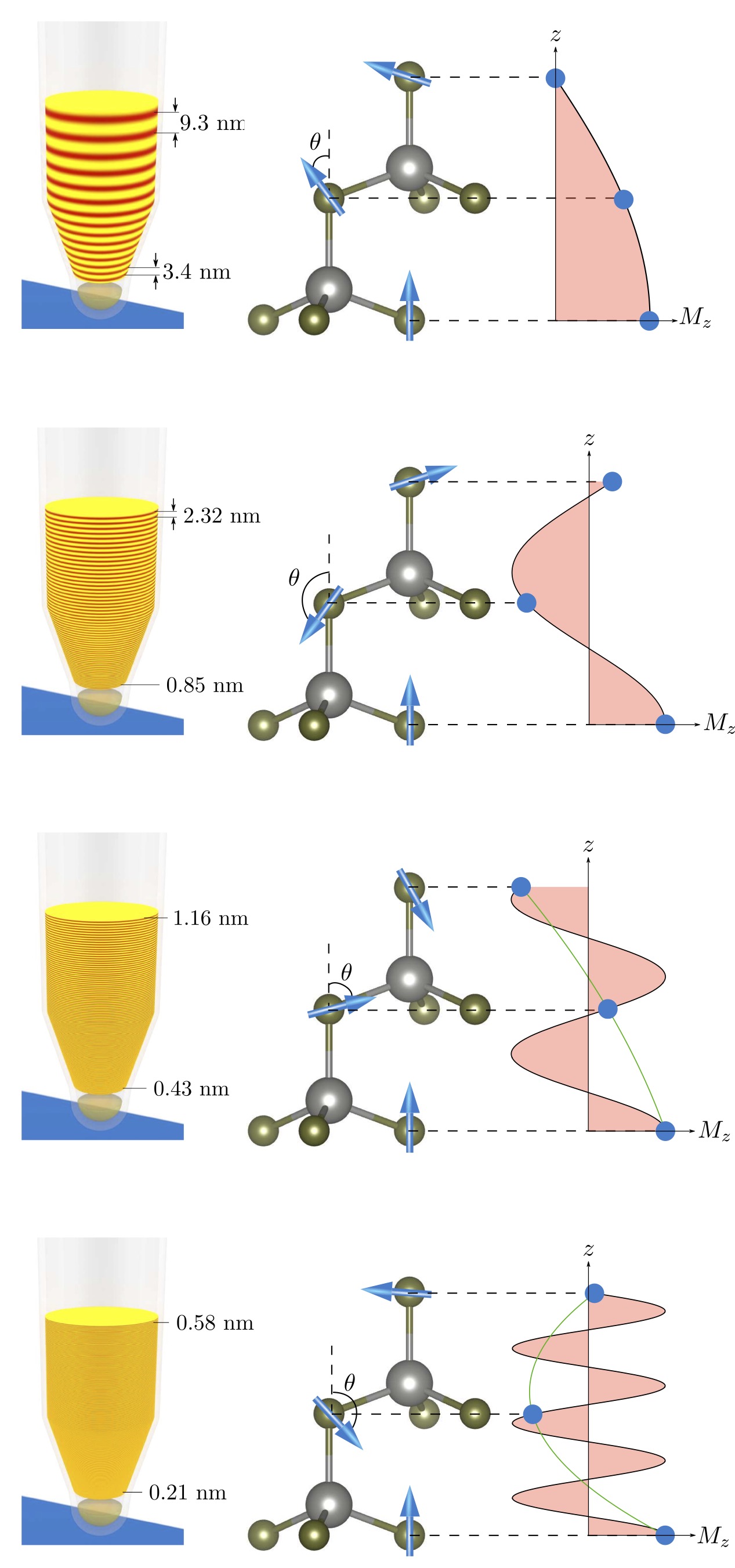
Along with these measurements, we have also demonstrated the capability to encode spin ensembles with magnetization profiles with pitches less than their lattice spacing. For the InP nanowires the lattice spacing along c-axis is 6.8 Å while we have shown the capability to encode spins with a pitch of 2.1 Å, albeit using a non-uniform spatial frequency.
These results were obtained with a field gradient device that generated non-uniform magnetic field gradients with which we only possessed the capability to encode the spin ensemble with a chirp modulation. In the upcoming spin transport experiments, we plan to measure the transport properties of the spin ensemble using next-generation field gradient source devices, which will allow us to encode with a sinusoidal modulation. In addition to InP, we are also looking to study Fluorapatite, a crystalline material known for its interesting many-body dynamics that resemble a 1D spin chain at short time scales.